Researchers Discover the Key to Bird Flight
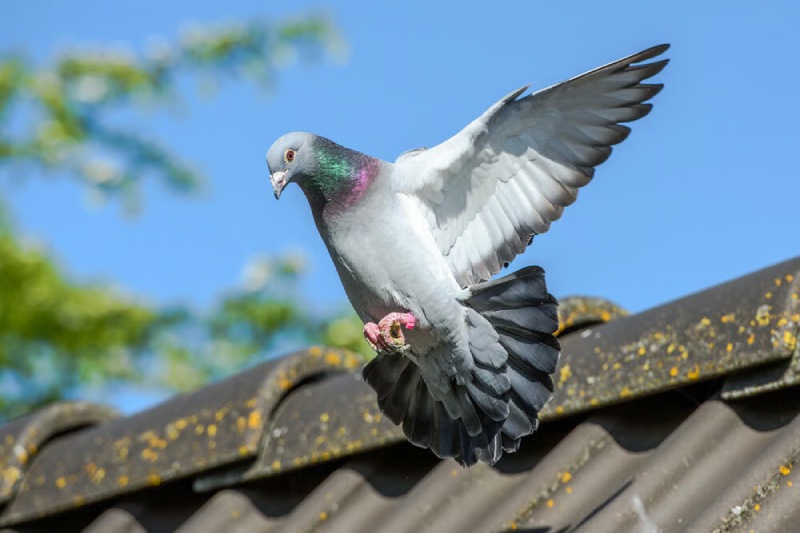
Birds are one of the most varied animal groups on the planet, with over 10,000 extant species and a wealth of fossil evidence demonstrating their evolutionary history. However, how did they become able to fly, a feat that is only possessed by two other species of vertebrates?
Evolutionary biologists at the Johns Hopkins University School of Medicine used data from studies of dinosaur fossils and pigeons using Positron Emission Tomography (PET), a nuclear medical imaging technique, to address an ongoing biological question: How did bird brains evolve to enable flight?
Their research indicates that the cerebellar portion of the brain, which is situated behind the brainstem and beneath the cerebrum, has grown in size in an adaptive manner. Among other things, this area is engaged in the development, learning, and planning of motor functions in fossilized vertebrates.
“Powered flight among vertebrates is a rare event in evolutionary history. just three groups of vertebrates, or animals with a backbone, evolved to fly: extinct pterosaurs – the terrors of the sky during the Mesozoic period, which ended over 65 million years ago, bats and birds,” said Dr. Amy Balanoff from the Johns Hopkins University School of Medicine.
Since the evolutionary lines of the three groups are not closely related, it is unknown what caused or how many variables allowed all three to fly. Apart from the anatomical modifications for flying, including the development of extended wings and specific kinds of feathers, Balanoff and her associates aimed to investigate the features of the brain that might support flying.
Balanoff worked with biomedical engineers at Stony Brook University in New York to compare the brain activity of contemporary pigeons prior to and following flight in order to achieve this goal.
In a study whose results were published in the Proceedings of the Royal Society B journal, the researchers compared activity in 26 brain regions in the birds during 10 seconds of flight versus rest using Positron Emission Tomography (PET), a technique also used to monitor metabolic processes in the human body.
PET scans employ a substance that resembles glucose to detect how it is absorbed by brain cells, revealing elevated activity and increased energy demand. Of the 26 locations, the cerebellum was the only one where all 10 birds studied had statistically significant increases in activity between rest and flight.
The optic flow pathways, a network of brain cells that connects the retina to the cerebellum and processes movement throughout the animal’s field of vision, were also shown to have higher levels of brain activity.
Balanoff said that while the cerebellum and optic flow pathways were already known to be important for flying, the results showing greater activity in these areas weren’t all that surprising.
The study did, however, also provide new insight into the relationship between the discovery that the cerebellum permits flight in modern birds and the documentation of bird fossils, which demonstrated the process by which avian ancestors resembling dinosaurs started to develop the brain conditions necessary for flight.
Utilizing a digital library of dinosaur endocasts—which, when filled, resemble brains—the researchers were able to accomplish this. They detected and monitored a discernible increase in the size of the cerebellum in some of the earliest dinosaurs belonging to the order Maniraptora, which predated dinosaurs with wings like the Archaeopteryx and is thought to be the closest relatives of contemporary birds.
The fact that the researchers employed a relatively straightforward flying path and an obstacle-free course for their investigation suggests that other brain regions may be more active during complex flight maneuvers.
Their current goal is to improve their understanding of the precise areas of the cerebellum that facilitate flight readiness, as well as the neurological connections among these components.
“At Johns Hopkins, the biomedical community has a wide-ranging set of tools and technology to help us understand evolutionary history and link our findings to fundamental research on how the brain works,” said Dr. Gabriel Bever from the School of Medicine at Johns Hopkins University.